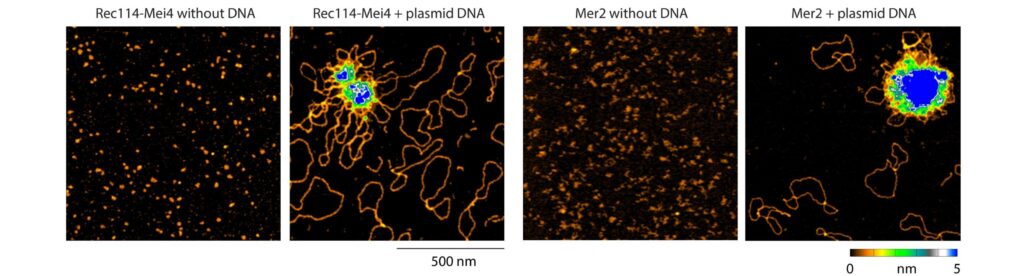
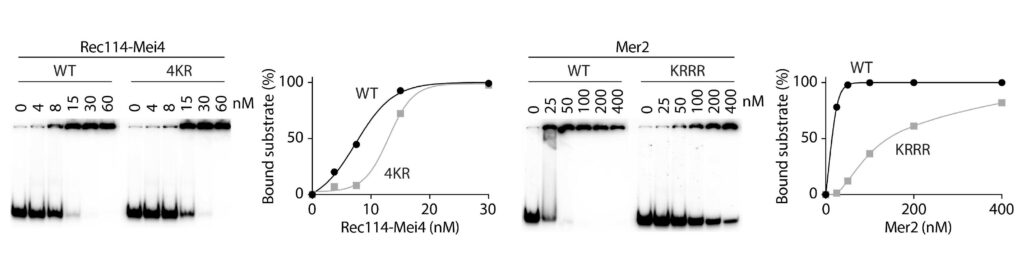
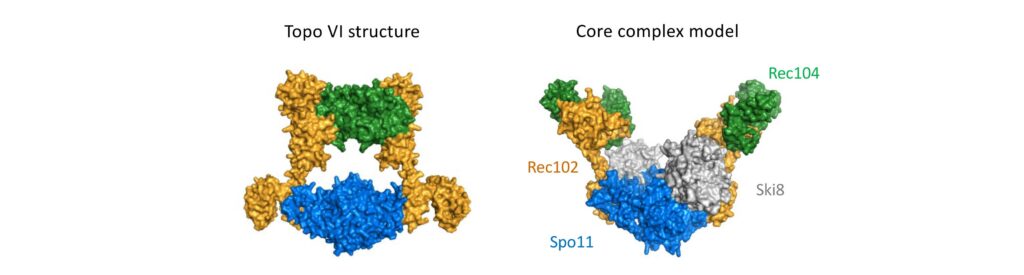
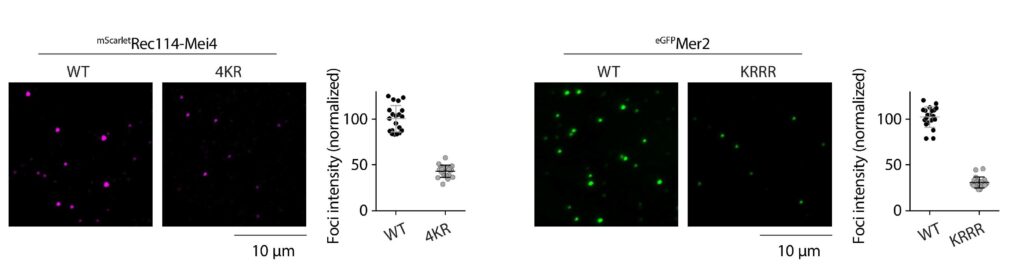
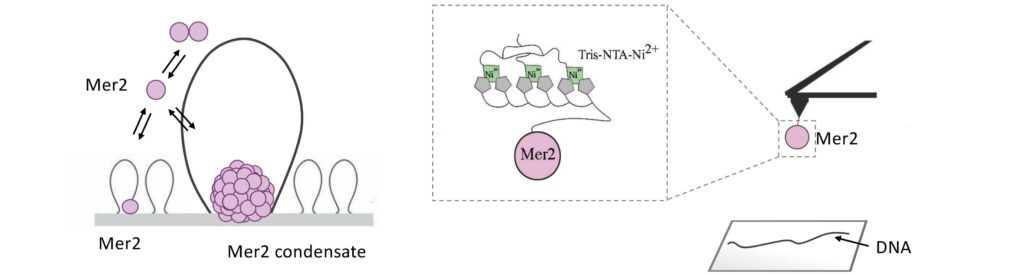
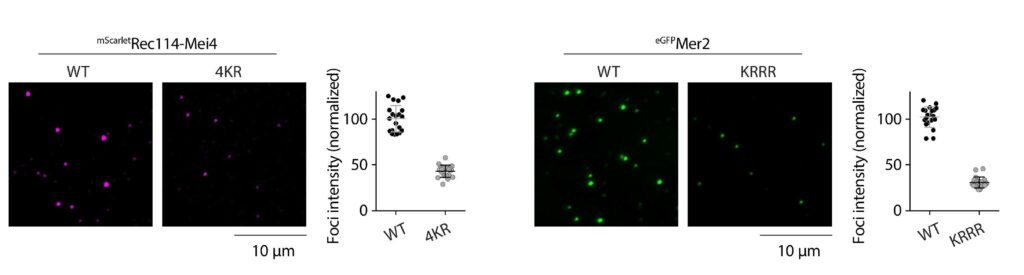
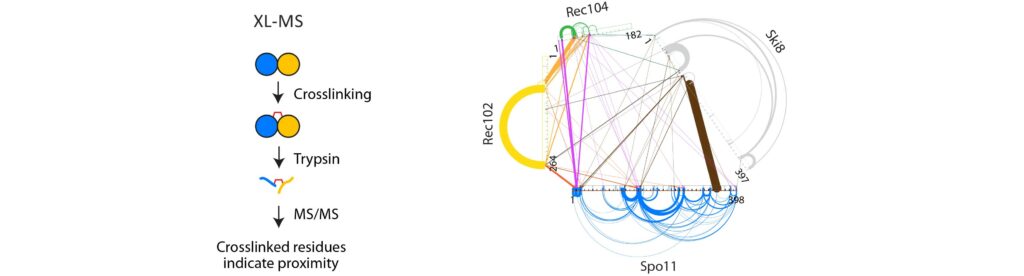
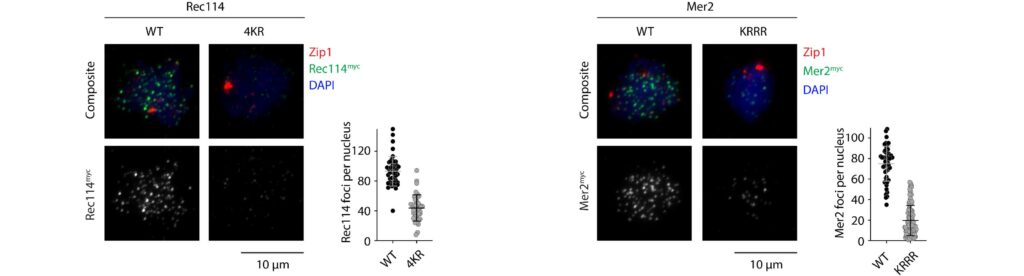
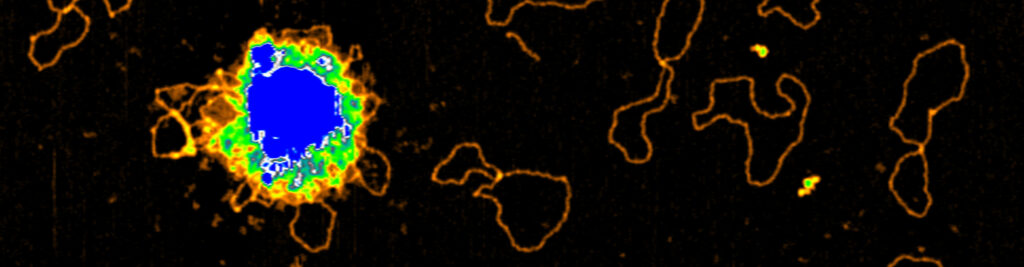
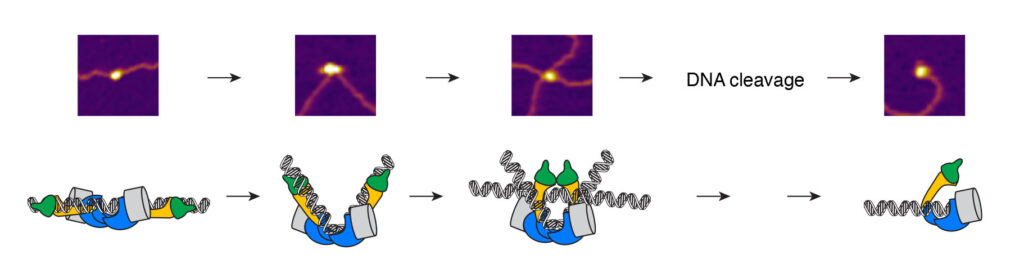
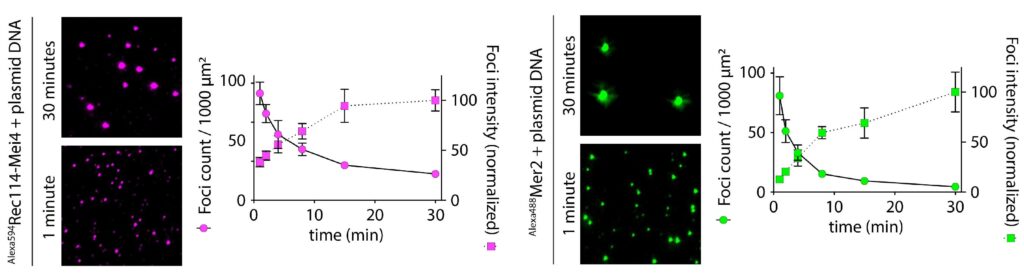
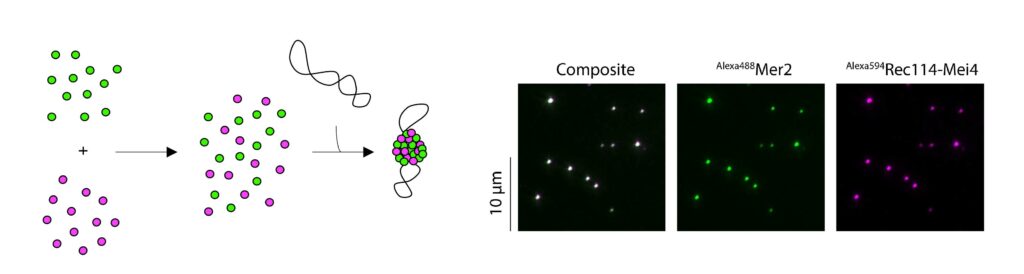
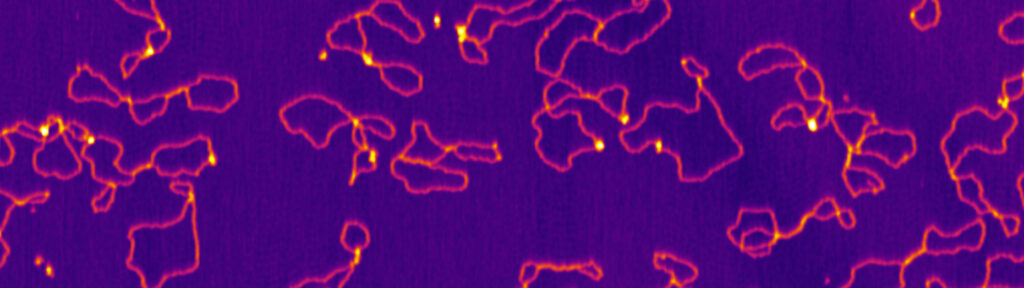
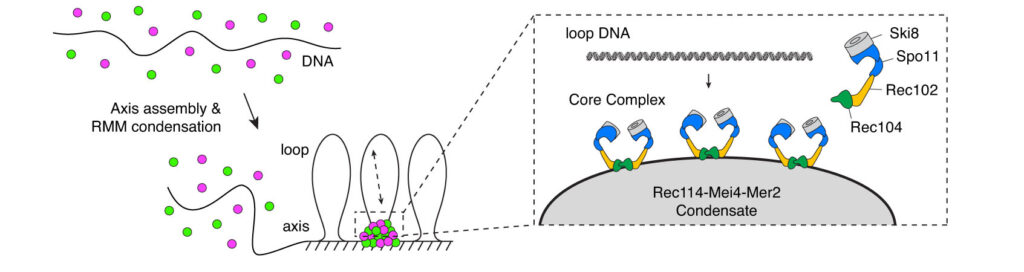
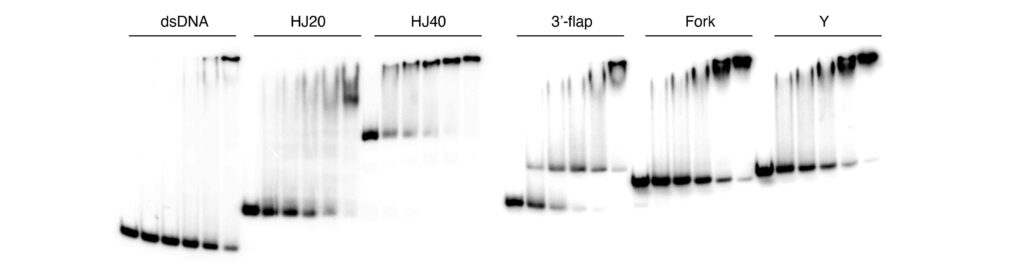
The mechanism of meiotic DNA double-strand break formation
Meiotic recombination starts when Spo11 makes DNA double-strand breaks (DSBs) throughout the genome. Spo11 evolved from the catalytic subunit of an archaeal type IIB topoisomerase (Topo VI). Two Spo11 subunits work in concert to make a DSB using an active-site tyrosine residue to attack the DNA backbone and generate a covalent 5′-phosphotyrosyl link (Figure 4A).
Breaking DNA is intrinsically dangerous. Indeed, DSBs are normally undesirable DNA damages that severely compromise cell physiology and survival. Yet meiotic cells induce high levels of breaks. Break formation is therefore controlled in terms of their number, timing and distribution. The cell induces DSB formation during a short window of time during the meiotic program, it dictates the formation of breaks at specific positions within the genome (called hotspots), and uses a series of regulatory feedback circuits to keep the number of breaks within an optimal range (about 200 per cell in yeast). However, how these multi-layered control mechanisms are integrated is not well understood.
Spo11 activity depends on at least nine co-factors (Figure 4B). These DSB proteins can be classified into three sub-complexes: The core complex that contains Spo11, the RMM complex, and the MRX complex. A central goal of our research is to understand how these proteins collaborate to catalyze DSB formation. We have isolated recombinant DSB proteins (Figure 4C) and are using in vitro methods to characterize their biochemical properties. We seek to gain insights into their molecular structure, to address how they interact with one another and with DNA, and, most importantly, to understand how the co-factors assist Spo11 in cleaving DNA.
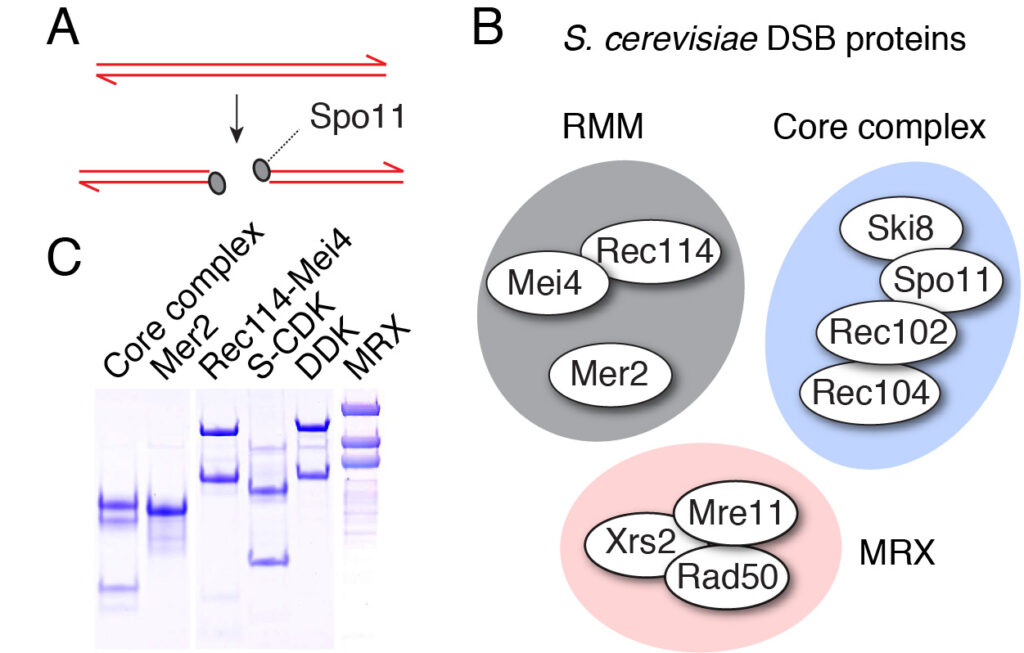
DSB formation and the organization of meiotic chromosomes
Meiotic chromosomes are arranged as a series of DNA loop anchored along a nucleoprotein axis (Figure 5A). DSB proteins are mostly located along the chromosome axis, but DNA cleavage happens preferentially within the loops. The tethered loop-axis model proposes a solution to this conundrum whereby a loop becomes tethered to the axis, allowing axis-bound Spo11 to cleave within the loop (Figure 5B). However, the underlying molecular assemblies remain poorly understood.
We have recently proposed that this assembly relies on the condensation of RMM along the chromosome. Our current model is that RMM condensates provide a platform that recruit the core complex and MRX (Figure 5C). The connection between the loop and the axis is then mediated by Spp1, that binds H3K4me3 methylation marks along the loop and axis-bound Mer2 proteins.
We aim to gain a better understanding of the relationship between DSB proteins and the chromosome axis. How do the DSB proteins become enriched along the axis? What are the molecular interactions that involved in the assembly of the DSB machinery?
