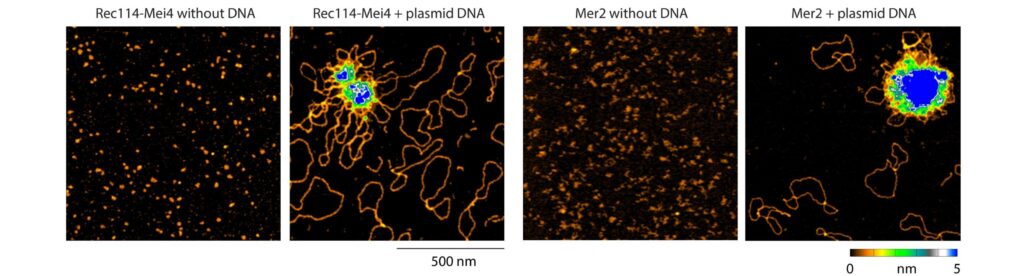
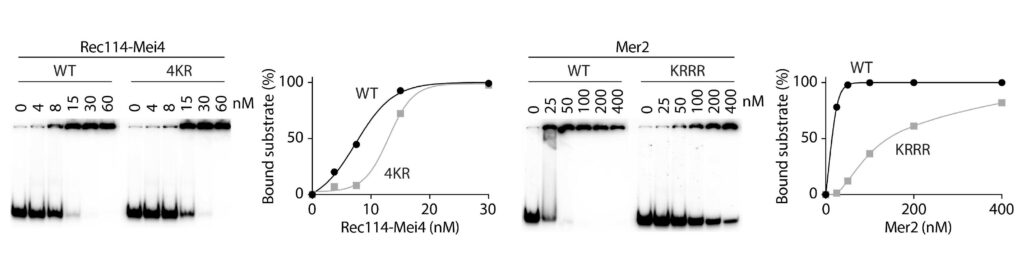
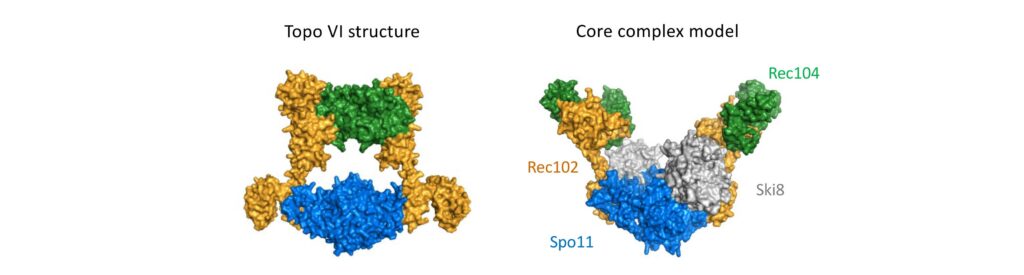
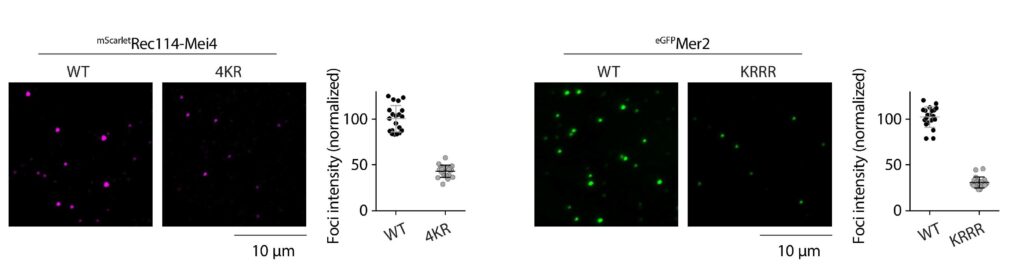
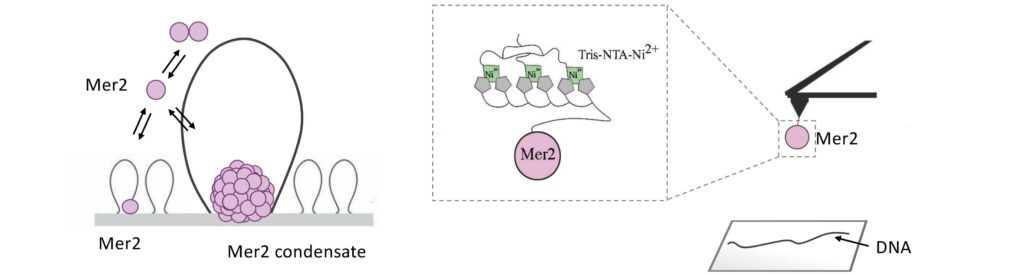
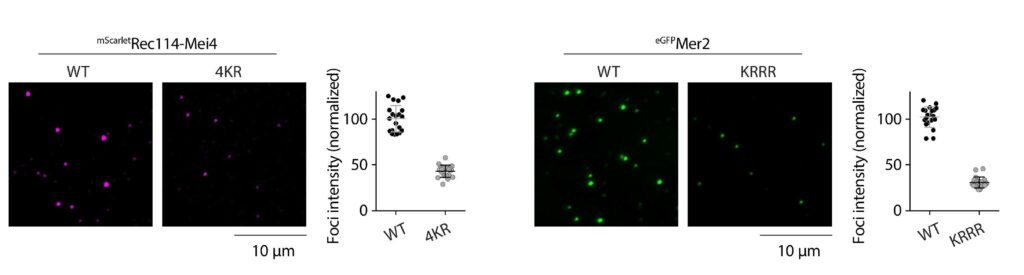
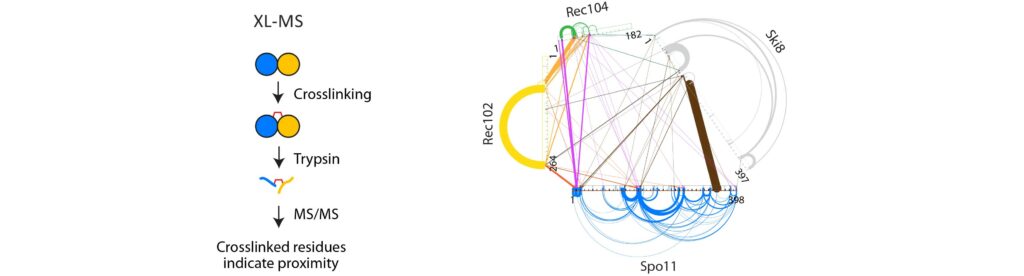
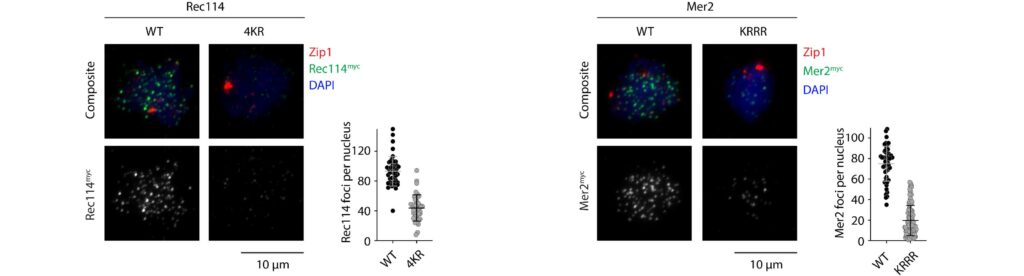
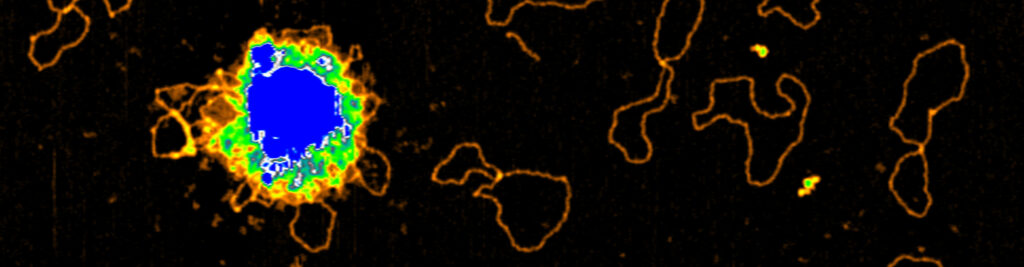
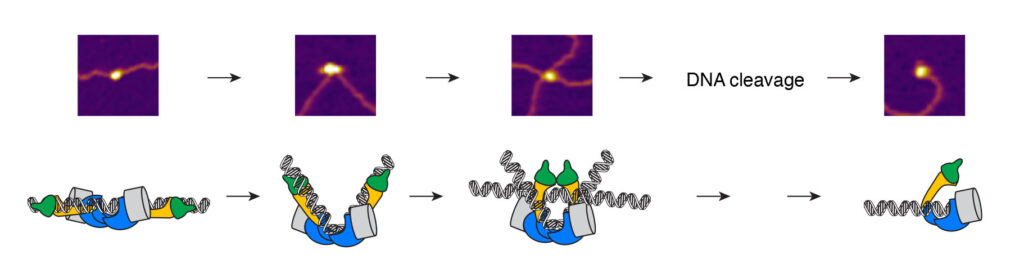
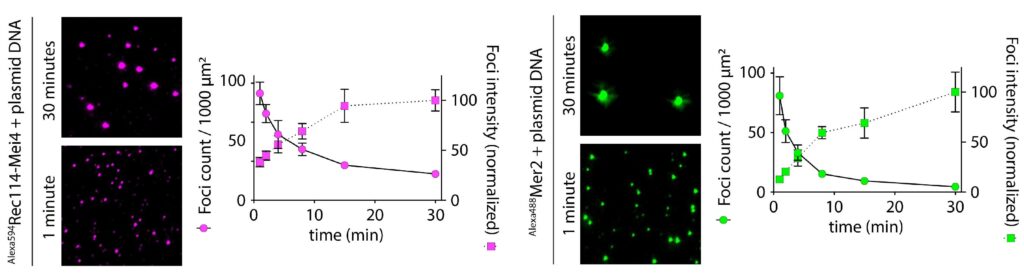
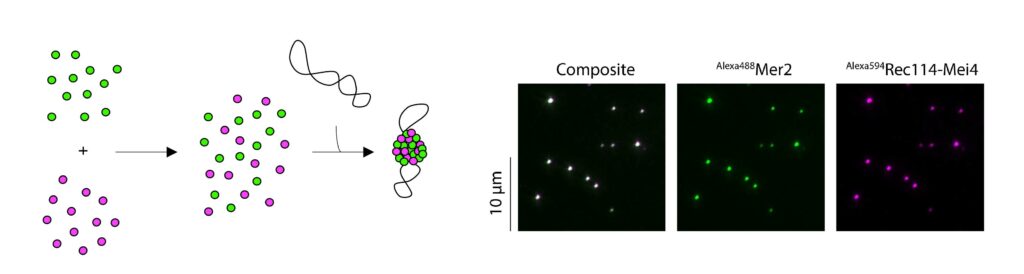
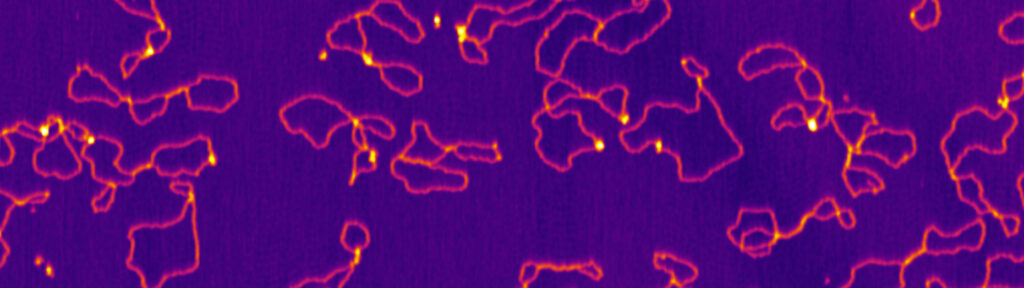
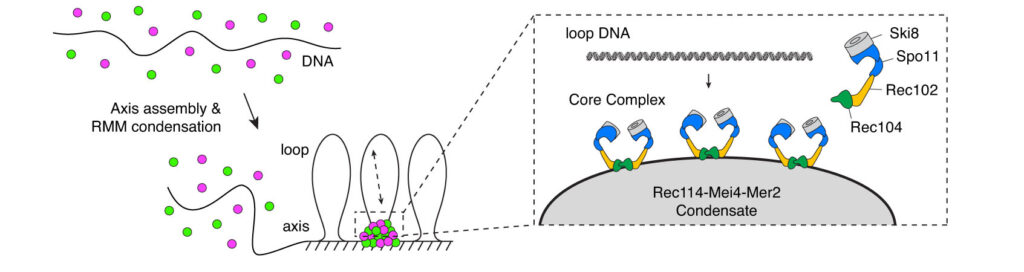
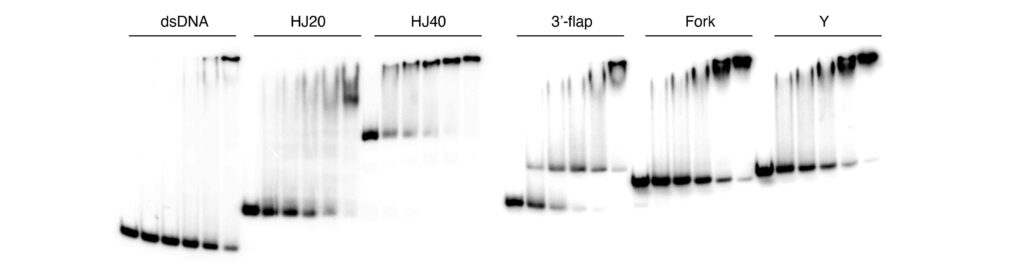
Meiosis and the inheritance of a unique genome
Sexual reproduction is an evolutionary successful strategy to promote genetic diversity. Indeed, most eukaryotes from single-cell fungi to humans reproduce sexually. By combining – and re-combining – genes from two individuals, each generation leads to new permutations of genes. Natural selection can then act to retain the best combinations, progressively driving evolution.
The number of chromosomes of an organism must remain constant from one generation to the next. Organisms therefore generate reproductive cells that have precisely half the number of chromosomes of regular cells, so that fecundation restores the normal number (Figure 1). This is the purpose of meiosis: to form haploid gametes with a single set of chromosomes (n) from a diploid progenitor (2n).
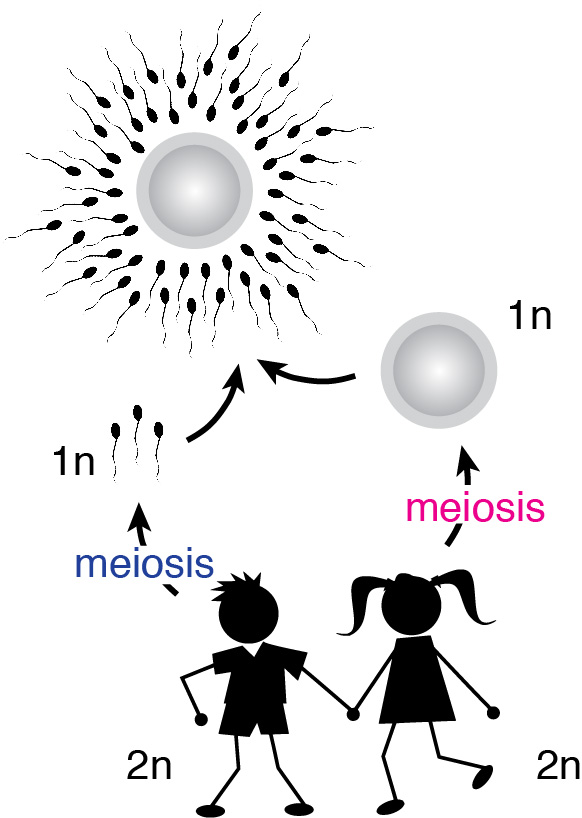
The cell does this by first duplicating its genome before dividing it twice in a row (Figure 2). However, meiotic cells face a challenge. Most cells have many chromosomes (23 pairs in humans, 16 in yeast), yet the gamete must inherit exactly one copy of each. So, how does the cell know which chromosomes are to be separated? Which chromosomes form pairs (i.e. are homologous)?
This involves a fascinating mechanism that is initiated by the programmed introduction of hundreds of DNA double-strand breaks, which will then be repaired by homologous recombination (Figure 3).
This allows the cell to search for DNA sequences that are identical (or highly similar) to the broken DNA sequence. When a repair template is found, the broken DNA sequence – that has now invaded the homolog and forms a recombination structure – copies information from the template. It is this recombination process, happening simultaneously throughout the genome, that allows homologous chromosomes to find each other within the cell. Progressively, the chromosomes will pair and then align along their entire length like a zipper, until all the chromosomes are fully synapsed.
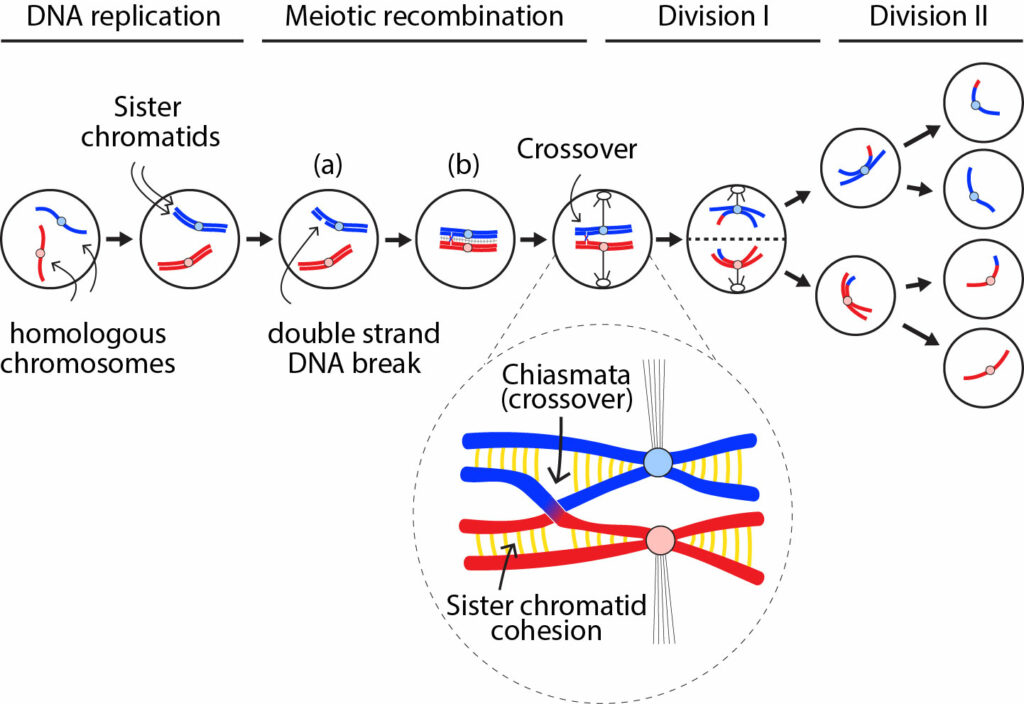
Finally, the last step of the recombination reaction takes place: the homologous chromosomes exchange DNA fragments to form crossovers (Figure 3). These crossovers serve a dual purpose: First, they serve to hold the homologous chromosomes together while they align along the meiotic spindle before their segregation (Figure 2, zoom). Second, crossovers generate new combinations of alleles, promoting genetic diversity. That is, crossovers are a central raison d’être of sexual reproduction.
We aim to understand two key aspects of meiosis: the formation of DNA double strand breaks and the formation of crossovers. We use yeast as a model organism because it is a convenient experimental system, and because the molecular processes involved in meiotic recombination are mostly conserved between yeast and humans. Our work is therefore relevant to human conditions related to genome integrity and chromosome biology, including cancer and fertility disorders.
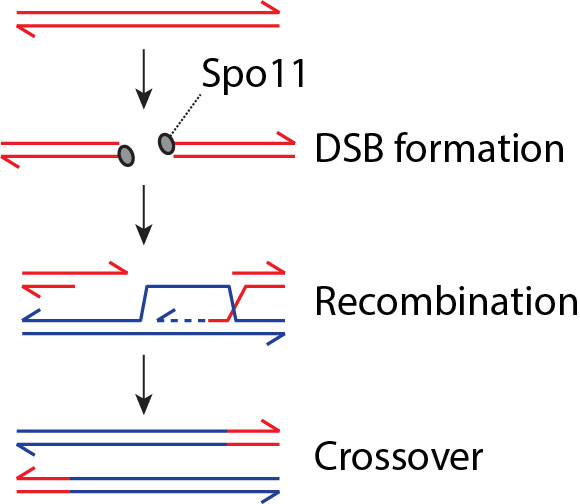